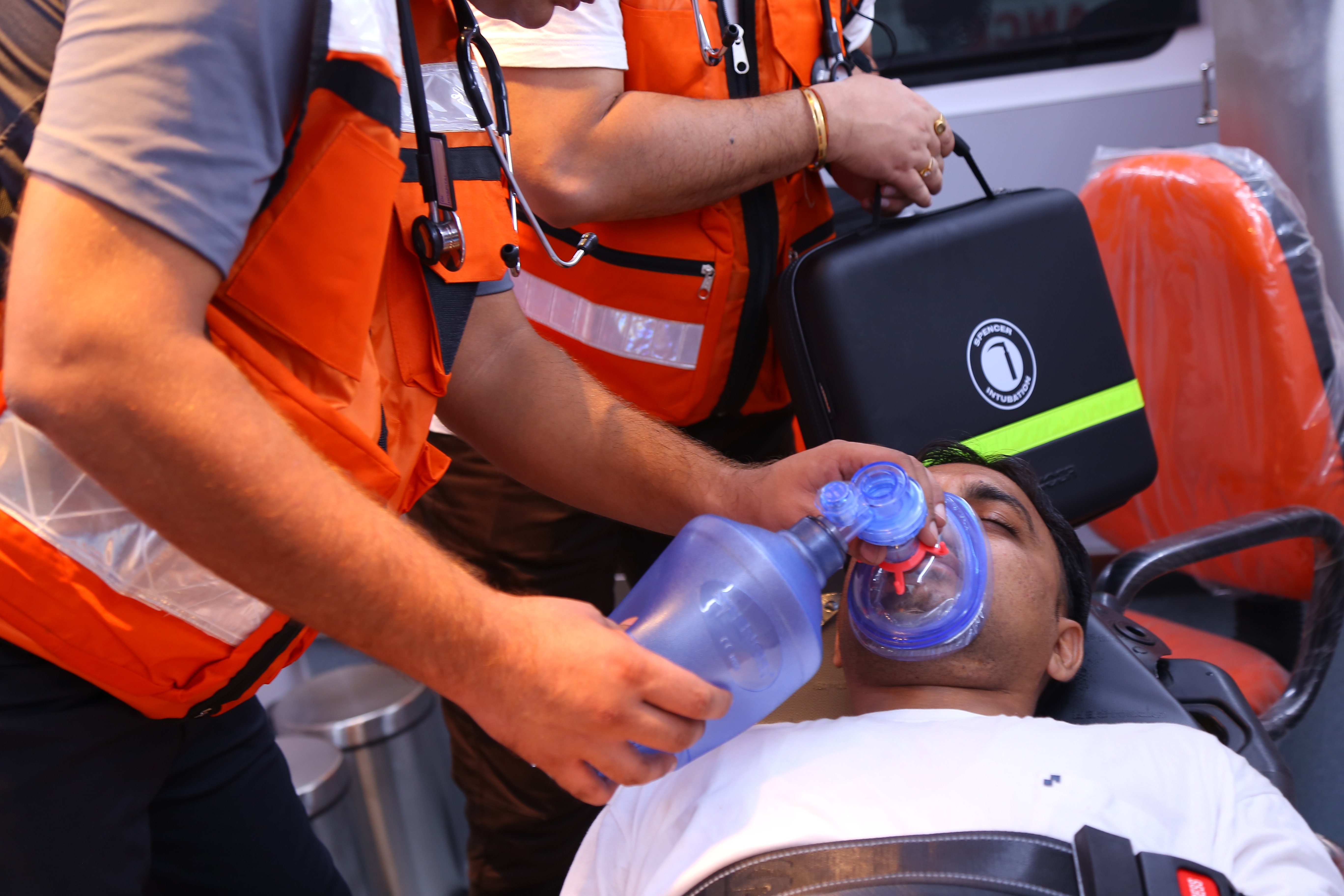
Ventilator management: ventilating the patient
Invasive mechanical ventilation is a frequently used intervention in acutely ill patients who require respiratory support or airway protection
The ventilator allows gas exchange to be maintained while other treatments are administered to improve clinical conditions
This activity reviews the indications, contraindications, management, and possible complications of invasive mechanical ventilation and emphasizes the importance of the interprofessional team in managing the care of patients requiring ventilatory support.
The need for mechanical ventilation is one of the most common causes of ICU admission.[1][2][3]
It is essential to understand some basic terms to understand mechanical ventilation
Ventilation: Exchange of air between the lungs and air (ambient or supplied by a ventilator), in other words, it is the process of moving air in and out of the lungs.
Its most important effect is the removal of carbon dioxide (CO2) from the body, not the increase of oxygen content in the blood.
In clinical settings, ventilation is measured as minute ventilation, calculated as respiratory rate (RR) times tidal volume (Vt).
In a mechanically ventilated patient, blood CO2 content can be changed by changing tidal volume or respiratory rate.
Oxygenation: Interventions that provide increased oxygen delivery to the lungs and thus to the circulation.
In a mechanically ventilated patient, this can be achieved by increasing the fraction of inspired oxygen (FiO 2%) or positive end-expiratory pressure (PEEP).
PEEP: The positive pressure remaining in the airway at the end of the respiratory cycle (end of expiration) is greater than atmospheric pressure in mechanically ventilated patients.
For a complete description of the use of PEEP, see the article entitled “Positive End-Expiratory Pressure (PEEP)” in the bibliographical references at the end of this article
Tidal volume: Volume of air moved in and out of the lungs in each respiratory cycle.
FiO2: Percentage of oxygen in the air mixture that is delivered to the patient.
Flow: Rate in liters per minute at which the ventilator delivers breaths.
Compliance: Change in volume divided by the change in pressure. In respiratory physiology, total compliance is a mixture of lung and chest wall compliance, since these two factors cannot be separated in a patient.
Because mechanical ventilation allows the physician to change the patient’s ventilation and oxygenation, it plays an important role in acute hypoxic and hypercapnic respiratory failure and severe acidosis or metabolic alkalosis.[4][5]
Physiology of mechanical ventilation
Mechanical ventilation has several effects on lung mechanics.
Normal respiratory physiology functions as a negative pressure system.
When the diaphragm pushes down during inspiration, negative pressure is generated in the pleural cavity, which, in turn, creates negative pressure in the airways that draw air into the lungs.
This same intrathoracic negative pressure decreases right atrial pressure (RA) and generates a suction effect on the inferior vena cava (IVC), increasing venous return.
The application of positive pressure ventilation modifies this physiology.
The positive pressure generated by the ventilator is transmitted to the upper airway and eventually to the alveoli; this, in turn, is transmitted to the alveolar space and the thoracic cavity, creating positive pressure (or at least lower negative pressure) in the pleural space.
The increase in RA pressure and the decrease in venous return generate a decrease in preload.
This has a dual effect of reducing cardiac output: less blood in the right ventricle means less blood reaches the left ventricle and less blood can be pumped out, reducing cardiac output.
A lower preload means that the heart is working at a less efficient point on the acceleration curve, generating less efficient work and further reducing cardiac output, which will result in a drop in mean arterial pressure (MAP) if there is no compensatory response through increased systemic vascular resistance (SVR).
This is a very important consideration in patients who may not be able to increase SVR, such as in patients with distributive shock (septic, neurogenic, or anaphylactic).
On the other hand, positive pressure mechanical ventilation can significantly reduce the work of breathing.
This, in turn, reduces blood flow to the respiratory muscles and redistributes it to the most critical organs.
Reducing the work of the respiratory muscles also reduces the generation of CO2 and lactate from these muscles, helping to improve acidosis.
The effects of positive pressure mechanical ventilation on venous return may be useful in patients with cardiogenic pulmonary edema
In these patients with volume overload, reducing venous return will directly decrease the amount of pulmonary edema generated, reducing right cardiac output.
At the same time, venous return reduction may improve left ventricular overdistension, placing it at a more advantageous point on the Frank-Starling curve and possibly improving cardiac output.
Proper management of mechanical ventilation also requires an understanding of pulmonary pressures and lung compliance.
Normal lung compliance is about 100 ml/cmH20.
This means that in a normal lung, administration of 500 ml of air by positive pressure ventilation will increase alveolar pressure by 5 cm H2O.
Conversely, administration of a positive pressure of 5 cm H2O will generate an increase in lung volume of 500 mL.
When working with abnormal lungs, compliance may be much higher or much lower.
Any disease that destroys lung parenchyma, such as emphysema, will increase compliance, while any disease that generates stiffer lungs (ARDS, pneumonia, pulmonary edema, pulmonary fibrosis) will decrease lung compliance.
The problem with rigid lungs is that small increases in volume can generate large increases in pressure and cause barotrauma.
This generates a problem in patients with hypercapnia or acidosis, as minute ventilation may need to be increased to correct these problems.
Increasing respiratory rate can manage this increase in minute ventilation, but if this is not feasible, increasing tidal volume can increase plateau pressures and create barotrauma.
There are two important pressures in the system to keep in mind when mechanically ventilating a patient:
- Peak pressure is the pressure reached during inspiration when air is pushed into the lungs and is a measure of airway resistance.
- Plateau pressure is the static pressure reached at the end of a full inspiration. To measure plateau pressure, an inspiratory pause must be performed on the ventilator to allow the pressure to equalize through the system. Plateau pressure is a measure of alveolar pressure and lung compliance. Normal plateau pressure is less than 30 cm H20, while higher pressure can generate barotrauma.
Indications for mechanical ventilation
The most common indication for intubation and mechanical ventilation is in cases of acute respiratory failure, either hypoxic or hypercapnic.
Other important indications are decreased level of consciousness with inability to protect the airway, respiratory distress that has failed noninvasive positive pressure ventilation, cases of massive hemoptysis, severe angioedema, or any case of airway compromise such as airway burns, cardiac arrest, and shock.
Common elective indications for mechanical ventilation are surgery and neuromuscular disorders.
Contraindications
There are no direct contraindications to mechanical ventilation, as it is a life-saving measure in a critically ill patient, and all patients should be offered the opportunity to benefit from it if necessary.
The only absolute contraindication to mechanical ventilation is if it is contrary to the patient’s stated desire for artificial life-sustaining measures.
The only relative contraindication is if noninvasive ventilation is available and its use is expected to resolve the need for mechanical ventilation.
This should be started first, as it has fewer complications than mechanical ventilation.
A number of steps should be taken to initiate mechanical ventilation
It is necessary to verify the correct placement of the endotracheal tube.
This can be done by end-tidal capnography or by a combination of clinical and radiological findings.
It is necessary to ensure adequate cardiovascular support with fluids or vasopressors, as indicated on a case-by-case basis.
Ensure that adequate sedation and analgesia are available.
The plastic tube in the patient’s throat is painful and uncomfortable, and if the patient is restless or struggles with the tube or ventilation, it will be much more difficult to control the different parameters of ventilation and oxygenation.
Ventilation modes
After intubating a patient and connecting him or her to the ventilator, it is time to select which ventilation mode to use.
In order to do this consistently for the benefit of the patient, several principles need to be understood.
As mentioned earlier, compliance is the change in volume divided by the change in pressure.
When mechanically ventilating a patient, you can choose how the ventilator will deliver breaths.
The ventilator can be set to deliver a predetermined amount of volume or a predetermined amount of pressure, and it is up to the physician to decide which is most beneficial to the patient.
When choosing ventilator delivery, we choose which will be the dependent variable and which will be the independent variable in the lung compliance equation.
If we choose to start the patient on volume-controlled ventilation, the ventilator will always deliver the same amount of volume (independent variable), while the pressure generated will depend on compliance.
If compliance is poor, the pressure will be high and barotrauma may occur.
On the other hand, if we decide to start the patient on pressure-controlled ventilation, the ventilator will always deliver the same pressure during the respiratory cycle.
However, the tidal volume will depend on lung compliance, and in cases where compliance changes frequently (as in asthma), this will generate unreliable tidal volumes and may cause hypercapnia or hyperventilation.
After selecting the mode of breath delivery (by pressure or volume), the physician must decide which ventilation mode to use.
This means choosing whether the ventilator will assist all of the patient’s breaths, some of the patient’s breaths, or none, and whether the ventilator will deliver breaths even if the patient is not breathing on his or her own.
Other parameters to consider are the rate of breath delivery (flow), the waveform of the flow (the decelerating waveform mimics physiological breaths and is more comfortable for the patient, while square waveforms, in which the flow is delivered at maximum rate throughout inspiration, are more uncomfortable for the patient but provide faster inhalation times), and the rate at which the breaths are delivered.
All these parameters must be adjusted to achieve patient comfort, desired blood gases, and avoid air entrapment.
There are several ventilation modes that vary minimally from each other. In this review we will focus on the most common ventilation modes and their clinical use.
Ventilation modes include assist control (AC), pressure support (PS), synchronized intermittent mandatory ventilation (SIMV), and airway pressure release ventilation (APRV).
Assisted ventilation (AC)
Assist control is where the ventilator assists the patient by providing support for each breath the patient takes (this is the assist part), while the ventilator has control over the respiratory rate if it falls below the set rate (control part).
In the assist control, if the frequency is set to 12 and the patient is breathing at 18, the ventilator will assist with the 18 breaths, but if the frequency drops to 8, the ventilator will take control of the respiratory rate and take 12 breaths per minute.
In assist-control ventilation, breaths can be delivered with either volume or pressure
This is referred to as volume-controlled ventilation or pressure-controlled ventilation.
To keep it simple and understand that since ventilation is commonly a more important issue than pressure and volume control is more commonly used than pressure control, for the remainder of this review we will use the term “volume control” interchangeably when talking about assist control.
The assist control (volume control) is the mode of choice used in most ICUs in the United States because it is easy to use.
Four settings (respiratory rate, tidal volume, FiO2, and PEEP) can be easily adjusted in the ventilator. The volume delivered by the ventilator in each breath in assisted control will always be the same, regardless of the breath initiated by the patient or ventilator and the compliance, peak or plateau pressures in the lungs.
Each breath can be timed (if the patient’s respiratory rate is lower than the ventilator’s setting, the machine will deliver breaths at a set interval) or triggered by the patient, in case the patient initiates a breath on his or her own.
This makes assistive control a very comfortable mode for the patient, as his or her every effort will be supplemented by the ventilator
After making changes to the ventilator or after starting a patient on mechanical ventilation, the arterial blood gases should be carefully checked and the oxygen saturation on the monitor should be followed to determine if any further changes need to be made to the ventilator.
The advantages of the AC mode are increased comfort, easy correction of respiratory acidosis/alkalosis, and low work of breathing for the patient.
Disadvantages include the fact that since this is a volume-cycle mode, pressures cannot be directly controlled, which can cause barotrauma, the patient may develop hyperventilation with breath stacking, autoPEEP, and respiratory alkalosis.
For a complete description of assisted control, see the article entitled “Ventilation, Assisted Control” [6], in the Bibliographical References part at the end of this article.
Synchronized Intermittent Mandatory Ventilation (SIMV)
SIMV is another frequently used ventilation modality, although its use has fallen into disuse due to less reliable tidal volumes and lack of better outcomes than AC.
“Synchronized” means that the ventilator adapts the delivery of its breaths to the patient’s efforts. “Intermittent” means that not all breaths are necessarily supported and “mandatory ventilation” means that, as in the case of CA, a predetermined frequency is selected and the ventilator delivers these mandatory breaths every minute regardless of the patient’s respiratory efforts.
The mandatory breaths can be triggered by patient or time if the patient’s RR is slower than the RR of the ventilator (as in the case of CA).
The difference from AC is that in SIMV the ventilator will deliver only the breaths that the frequency is set to deliver; any breaths taken by the patient above this frequency will not receive a tidal volume or full pressor support.
This means that for each breath taken by the patient above the set RR, the tidal volume delivered by the patient will depend solely on the patient’s lung compliance and effort.
This has been proposed as a method to “train” the diaphragm in order to maintain muscle tone and wean patients off the ventilator faster.
However, numerous studies have shown no benefit of SIMV. In addition, SIMV generates more respiratory work than AC, which has a negative impact on outcomes and generates respiratory fatigue.
A general rule of thumb to follow is that the patient will be released from the ventilator when he or she is ready, and no specific mode of ventilation will make it faster.
In the meantime, it is best to keep the patient as comfortable as possible, and SIMV may not be the best mode to achieve this.
Pressure Support Ventilation (PSV)
PSV is a ventilation mode that relies completely on patient-activated breaths.
As the name suggests, it is a pressure-driven ventilation mode.
In this mode, all breaths are initiated by the patient, as the ventilator has no backup rate, so each breath must be initiated by the patient. In this mode, the ventilator switches from one pressure to another (PEEP and support pressure).
PEEP is the pressure remaining at the end of exhalation, while pressure support is the pressure above PEEP that the ventilator will administer during each breath to sustain ventilation.
This means that if a patient is set in PSV 10/5, they will receive 5 cm H2O of PEEP and during inspiration they will receive 15 cm H2O of support (10 PS above PEEP).
Because there is no backup frequency, this mode cannot be used in patients with loss of consciousness, shock or cardiac arrest.
Current volumes depend solely on the patient’s exertion and lung compliance.
PSV is often used for weaning from the ventilator, as it merely increases the patient’s respiratory efforts without providing a predetermined tidal volume or respiratory rate.
The main disadvantage of PSV is the unreliability of tidal volume, which can generate CO2 retention and acidosis, and the high work of breathing that can lead to respiratory fatigue.
To solve this problem, a new algorithm was created for PSV, called volume-supported ventilation (VSV).
VSV is a mode similar to PSV, but in this mode the current volume is used as a feedback control, in that the pressor support provided to the patient is constantly adjusted according to the current volume. In this setting, if the tidal volume decreases, the ventilator will increase the pressor support to decrease the tidal volume, while if the tidal volume increases the pressor support will decrease to keep the tidal volume close to the desired minute ventilation.
Some evidence suggests that the use of VSV may reduce assisted ventilation time, total weaning time and total T-piece time, as well as decrease the need for sedation.
Airway pressure release ventilation (APRV)
As the name suggests, in the APRV mode, the ventilator delivers a constant high pressure in the airway, which ensures oxygenation, and ventilation is performed by releasing this pressure.
This mode has recently gained popularity as an alternative for patients with ARDS who are difficult to oxygenate, in whom other ventilation modes fail to achieve their goals.
APRV has been described as continuous positive airway pressure (CPAP) with an intermittent release phase.
This means that the ventilator applies a continuous high pressure (P high) for a set period of time (T high) and then releases it, usually returning to zero (P low) for a much shorter period of time (T low).
The idea behind this is that during T high (covering 80%-95% of the cycle), there is constant alveolar recruitment, which improves oxygenation because the time maintained at high pressure is much longer than during other types of ventilation (open lung strategy).
This reduces the repetitive inflation and deflation of the lungs that occurs with other modes of ventilation, preventing ventilator-induced lung injury.
During this period (T high) the patient is free to breathe spontaneously (which makes him or her comfortable), but will pull low tidal volumes because exhaling against such pressure is more difficult. Then, when T high is reached, the pressure in the ventilator drops to P low (usually zero).
Air is then expelled from the airway, allowing passive exhalation until T low is reached and the ventilator delivers another breath.
To prevent airway collapse during this period, the low T is set briefly, usually around 0.4-0.8 seconds.
In this case, when the ventilator pressure is set to zero, the elastic recoil of the lungs pushes air outward, but the time is not long enough to get all the air out of the lungs, so the alveolar and airway pressures do not reach zero and airway collapse does not occur.
This time is usually set so that the low T ends when the exhalation flow drops to 50% of the initial flow.
The ventilation per minute, therefore, will depend on the T low and the patient’s tidal volume during the T high
Indications for the use of APRV:
- ARDS difficult to oxygenate with AC
- Acute lung injury
- Postoperative atelectasis.
Advantages of APRV:
APRV is a good modality for lung protective ventilation.
The ability to set a high P means that the operator has control over the plateau pressure, which can significantly reduce the incidence of barotrauma.
As the patient begins his or her respiratory efforts, there is better gas distribution due to a better V/Q match.
Constant high pressure means increased recruitment (open lung strategy).
APRV can improve oxygenation in patients with ARDS who are difficult to oxygenate with AC.
APRV may reduce the need for sedation and neuromuscular blocking agents, as the patient may be more comfortable compared with other modalities.
Disadvantages and contraindications:
Because spontaneous breathing is an important aspect of APRV, it is not ideal for heavily sedated patients.
There are no data on the use of APRV in neuromuscular disorders or obstructive lung disease, and its use should be avoided in these patient populations.
Theoretically, constant high intrathoracic pressure could generate elevated pulmonary artery pressure and worsen intracardiac shunts in patients with Eisenmenger’s physiology.
Strong clinical reasoning is needed when choosing APRV as a mode of ventilation over more conventional modes such as AC.
Further information on the details of the different ventilation modes and their setting can be found in the articles on each specific ventilation mode.
Use of the ventilator
The initial setting of the ventilator can vary greatly depending on the cause of intubation and the purpose of this review.
However, there are some basic settings for most cases.
The most common ventilator mode to use in a newly intubated patient is AC mode.
The AC mode provides good comfort and easy control of some of the most important physiological parameters.
It starts with a FiO2 of 100% and decreases guided by pulse oximetry or ABG, as appropriate.
Low tidal volume ventilation has been shown to be lung protective not only in ARDS but also in other types of diseases.
Starting the patient with a low tidal volume (6 to 8 mL/Kg ideal body weight) reduces the incidence of ventilator-induced lung injury (VILI).
Always use a lung protection strategy, as higher tidal volumes have little benefit and increase shear stress in the alveoli and may induce lung injury.
The initial RR should be comfortable for the patient: 10-12 bpm is sufficient.
A very important caveat concerns patients with severe metabolic acidosis.
For these patients, ventilation per minute must at least match pre-intubation ventilation, as otherwise acidosis worsens and may precipitate complications such as cardiac arrest.
Flow should be initiated at or above 60 L/min to avoid autoPEEP
Start with a low PEEP of 5 cm H2O and increase according to the patient’s tolerance to the oxygenation goal.
Pay close attention to blood pressure and patient comfort.
An ABG should be obtained 30 min after intubation and ventilator settings should be adjusted according to ABG results.
Peak and plateau pressures should be checked on the ventilator to make sure there are no problems with airway resistance or alveolar pressure to prevent ventilator-induced lung damage.
Attention should be paid to the volume curves on the ventilator display, as a reading showing that the curve does not return to zero upon exhalation is indicative of incomplete exhalation and the development of auto-PEEP; therefore, corrections should be made to the ventilator immediately.[7][8]
Ventilator troubleshooting
With a good understanding of the concepts discussed, managing ventilator complications and troubleshooting should become second nature.
The most common corrections to be made to ventilation involve hypoxemia and hypercapnia or hyperventilation:
Hypoxia: oxygenation depends on FiO2 and PEEP (high T and high P for APRV).
To correct hypoxia, increasing either of these parameters should increase oxygenation.
Special attention should be paid to the possible adverse effects of increasing PEEP, which can cause barotrauma and hypotension.
Increasing FiO2 is not without concern, as elevated FiO2 can cause oxidative damage in the alveoli.
Another important aspect of oxygen content management is setting an oxygenation goal.
In general, it is of little benefit to maintain oxygen saturation above 92-94%, except, for example, in cases of carbon monoxide poisoning.
A sudden drop in oxygen saturation should raise suspicion of tube malpositioning, pulmonary embolism, pneumothorax, pulmonary edema, atelectasis, or development of mucus plugs.
Hypercapnia: To change the blood CO2 content, alveolar ventilation must be modified.
This can be done by altering tidal volume or respiratory rate (low T and low P in APRV).
Increasing the rate or tidal volume, as well as increasing T low, increases ventilation and reduces CO2.
Care must be taken with increasing frequency, as it will also increase the amount of dead space and may not be as effective as tidal volume.
When increasing volume or frequency, special attention must be paid to the flow-volume loop to avoid the development of auto-PEEP.
High pressures: Two pressures are important in the system: peak pressure and plateau pressure.
Peak pressure is a measure of airway resistance and compliance and includes the tube and bronchial tree.
Plateau pressures reflect alveolar pressure and thus lung compliance.
If there is an increase in peak pressure, the first step is to take an inspiratory pause and check the plateau.
High peak pressure and normal plateau pressure: high airway resistance and normal compliance
Possible causes: (1) Twisted ET tube-The solution is to untwist the tube; use a bite lock if the patient bites the tube, (2) Mucus plug-The solution is to aspirate the patient, (3) Bronchospasm-The solution is to administer bronchodilators.
High peak and high plateau: Compliance problems
Possible causes include:
- Main trunk intubation-The solution is to retract the ET tube. For diagnosis, you will find a patient with unilateral breath sounds and a contralateral lung off (atelectatic lung).
- Pneumothorax: Diagnosis will be made by listening to breath sounds unilaterally and finding a contralateral hyperresonant lung. In intubated patients, placement of a chest tube is imperative, as positive pressure will only worsen the pneumothorax.
- Atelectasis: Initial management consists of chest percussion and recruitment maneuvers. Bronchoscopy may be used in resistant cases.
- Pulmonary edema: Diuresis, inotropes, elevated PEEP.
- ARDS: Use low tidal volume and high PEEP ventilation.
- Dynamic hyperinflation or auto-PEEP: is a process in which some of the inhaled air is not fully exhaled at the end of the respiratory cycle.
- The accumulation of trapped air increases lung pressures and causes barotrauma and hypotension.
- The patient will be difficult to ventilate.
- To prevent and resolve self-PEEP, sufficient time must be allowed for air to leave the lungs during exhalation.
The goal in management is to decrease the inspiratory/expiratory ratio; this can be achieved by decreasing the respiratory rate, decreasing tidal volume (a higher volume will require a longer time to leave the lungs), and increasing inspiratory flow (if air is delivered rapidly, the inspiratory time is shorter and the expiratory time will be longer at any respiratory rate).
The same effect can be achieved by using a square waveform for inspiratory flow; this means that we can set the ventilator to deliver the entire flow from the beginning to the end of inspiration.
Other techniques that can be put in place are ensuring adequate sedation to prevent hyperventilation of the patient and the use of bronchodilators and steroids to reduce airway obstruction.
If auto-PEEP is severe and causes hypotension, disconnecting the patient from the ventilator and allowing all air to be exhaled may be a life-saving measure.
For a complete description of the management of auto-PEEP, see the article titled “Positive End-Expiratory Pressure (PEEP).”
Another common problem encountered in patients undergoing mechanical ventilation is patient-ventilator dyssynchrony, usually referred to as “ventilator struggle.”
Important causes include hypoxia, self-PEEP, failure to meet the patient’s oxygenation or ventilation requirements, pain, and discomfort.
After ruling out important causes such as pneumothorax or atelectasis, consider patient comfort and ensure adequate sedation and analgesia.
Consider changing the ventilation mode, as some patients may respond better to different ventilation modes.
Special attention should be paid to ventilation settings under the following circumstances:
- COPD is a special case, as pure COPD lungs have high compliance, which causes a high tendency for dynamic airflow obstruction due to airway collapse and air entrapment, making COPD patients very prone to develop auto-PEEP. Using a preventive ventilation strategy with high flow and low respiratory rate can help prevent self-PEEP. Another important aspect to consider in chronic hypercapnic respiratory failure (due to COPD or another reason) is that it is not necessary to correct CO2 to bring it back to normal, as these patients usually have metabolic compensation for their respiratory problems. If a patient is ventilated to normal CO2 levels, his bicarbonate decreases and, when extubated, he quickly goes into respiratory acidosis because the kidneys cannot respond as quickly as the lungs and CO2 returns to baseline, causing respiratory failure and reintubation. To avoid this, CO2 targets must be determined based on pH and the previously known or calculated baseline.
- Asthma: As with COPD, patients with asthma are very prone to air entrapment, although the reason is pathophysiologically different. In asthma, air entrapment is caused by inflammation, bronchospasm and mucus plugs, not airway collapse. The strategy to prevent self-PEEP is similar to that used in COPD.
- Cardiogenic pulmonary edema: elevated PEEP can decrease venous return and help resolve pulmonary edema, as well as promote cardiac output. The concern should be to ensure that the patient is adequately diuretic before extubating, as removal of positive pressure may precipitate new pulmonary edema.
- ARDS is a type of noncardiogenic pulmonary edema. An open lung strategy with high PEEP and low tidal volume has been shown to improve mortality.
- Pulmonary embolism is a difficult situation. These patients are very preload-dependent because of the acute rise in right atrial pressure. Intubation of these patients will increase RA pressure and further reduce venous return, with the risk of precipitating shock. If there is no way to avoid intubation, attention should be paid to blood pressure and vasopressor administration should be started promptly.
- Severe pure metabolic acidosis is a problem. When intubating these patients, close attention should be paid to their minute pre-intubation ventilation. If this ventilation is not provided when mechanical support is started, the pH will drop further, which may precipitate cardiac arrest.
Bibliographic references
- Metersky ML, Kalil AC. Management of Ventilator-Associated Pneumonia: Guidelines. Clin Chest Med. 2018 Dec;39(4):797-808. [PubMed]
- Chomton M, Brossier D, Sauthier M, Vallières E, Dubois J, Emeriaud G, Jouvet P. Ventilator-Associated Pneumonia and Events in Pediatric Intensive Care: A Single Center Study. Pediatr Crit Care Med. 2018 Dec;19(12):1106-1113. [PubMed]
- Vandana Kalwaje E, Rello J. Management of ventilator-associated pneumonia: Need for a personalized approach. Expert Rev Anti Infect Ther. 2018 Aug;16(8):641-653. [PubMed]
- Jansson MM, Syrjälä HP, Talman K, Meriläinen MH, Ala-Kokko TI. Critical care nurses’ knowledge of, adherence to, and barriers toward institution-specific ventilator bundle. Am J Infect Control. 2018 Sep;46(9):1051-1056. [PubMed]
- Piraino T, Fan E. Acute life-threatening hypoxemia during mechanical ventilation. Curr Opin Crit Care. 2017 Dec;23(6):541-548. [PubMed]
- Mora Carpio AL, Mora JI. StatPearls [Internet]. StatPearls Publishing; Treasure Island (FL): Apr 28, 2022. Ventilation Assist Control. [PubMed]
- Kumar ST, Yassin A, Bhowmick T, Dixit D. Recommendations From the 2016 Guidelines for the Management of Adults With Hospital-Acquired or Ventilator-Associated Pneumonia. P T. 2017 Dec;42(12):767-772. [PMC free article] [PubMed]
- Del Sorbo L, Goligher EC, McAuley DF, Rubenfeld GD, Brochard LJ, Gattinoni L, Slutsky AS, Fan E. Mechanical Ventilation in Adults with Acute Respiratory Distress Syndrome. Summary of the Experimental Evidence for the Clinical Practice Guideline. Ann Am Thorac Soc. 2017 Oct;14(Supplement_4):S261-S270. [PubMed]
- Chao CM, Lai CC, Chan KS, Cheng KC, Ho CH, Chen CM, Chou W. Multidisciplinary interventions and continuous quality improvement to reduce unplanned extubation in adult intensive care units: A 15-year experience. Medicine (Baltimore). 2017 Jul;96(27):e6877. [PMC free article] [PubMed]
- Badnjevic A, Gurbeta L, Jimenez ER, Iadanza E. Testing of mechanical ventilators and infant incubators in healthcare institutions. Technol Health Care. 2017;25(2):237-250. [PubMed]
Read Also
Emergency Live Even More…Live: Download The New Free App Of Your Newspaper For IOS And Android
Three Everyday Practices To Keep Your Ventilator Patients Safe
Ambulance: What Is An Emergency Aspirator And When Should It Be Used?
The Purpose Of Suctioning Patients During Sedation
Supplemental Oxygen: Cylinders And Ventilation Supports In The USA
Basic Airway Assessment: An Overview
Respiratory Distress: What Are The Signs Of Respiratory Distress In Newborns?
EDU: Directional Tip Suction Catheter
Suction Unit For Emergency Care, The Solution In A Nutshell: Spencer JET
Airway Management After A Road Accident: An Overview
Tracheal Intubation: When, How And Why To Create An Artificial Airway For The Patient
What Is Transient Tachypnoea Of The Newborn, Or Neonatal Wet Lung Syndrome?
Traumatic Pneumothorax: Symptoms, Diagnosis And Treatment
Diagnosis Of Tension Pneumothorax In The Field: Suction Or Blowing?
Pneumothorax And Pneumomediastinum: Rescuing The Patient With Pulmonary Barotrauma
ABC, ABCD And ABCDE Rule In Emergency Medicine: What The Rescuer Must Do
Multiple Rib Fracture, Flail Chest (Rib Volet) And Pneumothorax: An Overview
Internal Haemorrhage: Definition, Causes, Symptoms, Diagnosis, Severity, Treatment
Assessment Of Ventilation, Respiration, And Oxygenation (Breathing)
Oxygen-Ozone Therapy: For Which Pathologies Is It Indicated?
Difference Between Mechanical Ventilation And Oxygen Therapy
Hyperbaric Oxygen In The Wound Healing Process
Venous Thrombosis: From Symptoms To New Drugs
What Is Intravenous Cannulation (IV)? The 15 Steps Of The Procedure
Nasal Cannula For Oxygen Therapy: What It Is, How It Is Made, When To Use It
Nasal Probe For Oxygen Therapy: What It Is, How It Is Made, When To Use It
Oxygen Reducer: Principle Of Operation, Application
How To Choose Medical Suction Device?
Holter Monitor: How Does It Work And When Is It Needed?
What Is Patient Pressure Management? An Overview
Head Up Tilt Test, How The Test That Investigates The Causes Of Vagal Syncope Works
Cardiac Syncope: What It Is, How It Is Diagnosed And Who It Affects
Cardiac Holter, The Characteristics Of The 24-Hour Electrocardiogram