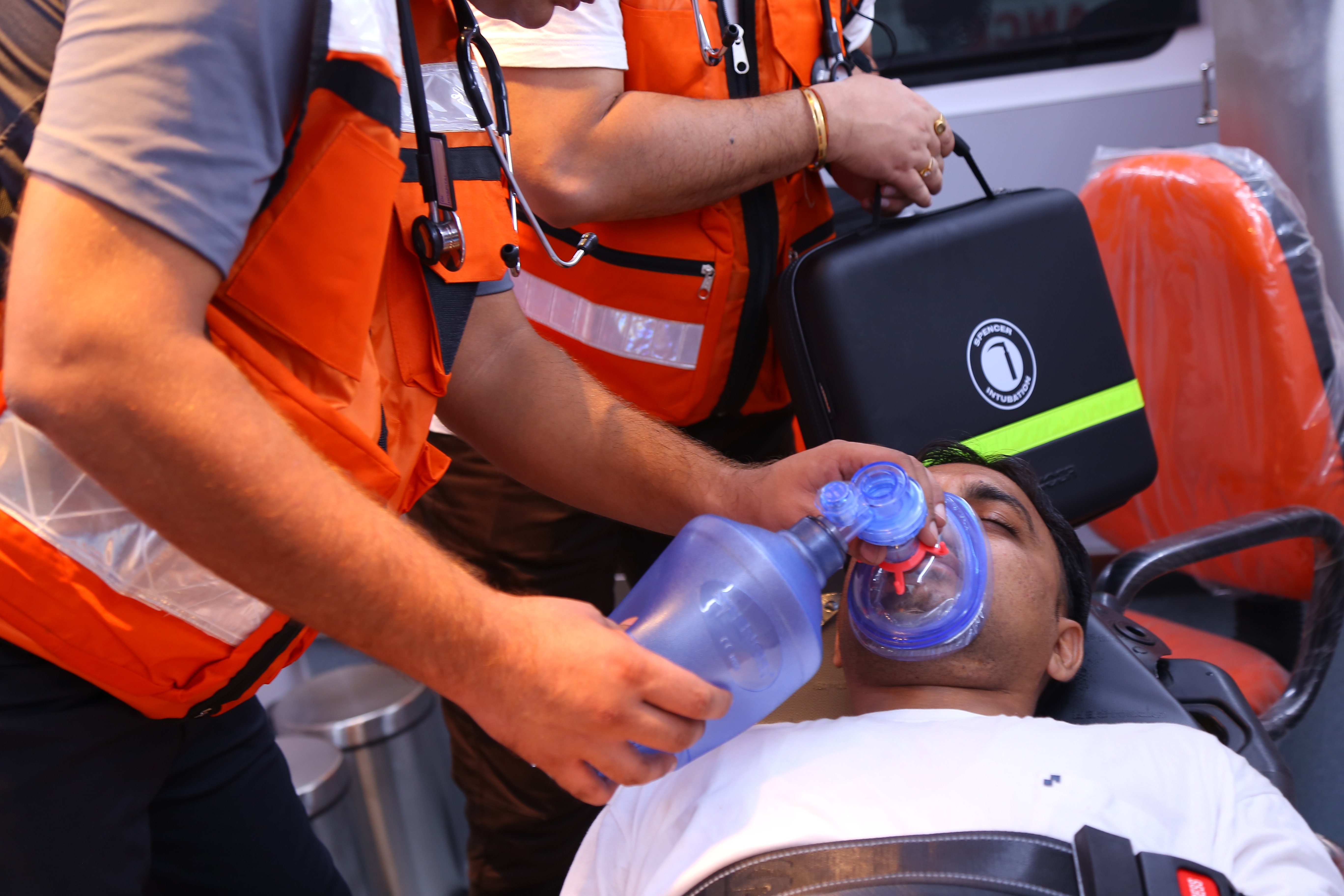
Respiratory Distress Syndrome (ARDS): therapy, mechanical ventilation, monitoring
Acute respiratory distress syndrome (hence the acronym ‘ARDS’) is a respiratory pathology caused by various causes and characterised by diffuse damage to the alveolar capillaries leading to severe respiratory failure with arterial hypoxaemia refractory to oxygen administration
ARDS is thus characterised by a decrease in the concentration of oxygen in the blood, which is resistant to O2 therapy, i.e. this concentration does not rise following the administration of oxygen to the patient.
Hypoxaemic respiratory failure is due to a lesion of the alveolar-capillary membrane, which increases pulmonary vascular permeability, leading to interstitial and alveolar oedema.
The treatment of ARDS is, fundamentally, supportive and consists of
- treatment of the upstream cause that triggered ARDS;
- maintenance of adequate tissue oxygenation (ventilation and cardiopulmonary assistance);
- nutritional support.
ARDS is a syndrome triggered by many different precipitating factors leading to similar lung damage
On some of the causes of ARDS it is not possible to intervene, but in cases where this is feasible (such as in the case of shock or sepsis), early and effective treatment becomes crucial to limit the severity of the syndrome and to increase the patient’s chances of survival.
Pharmacological treatment of ARDS is aimed at correcting the underlying disorders and providing support for cardiovascular function (e.g. antibiotics to treat infection and vasopressors to treat hypotension).
Tissue oxygenation depends on adequate oxygen release (O2del), which is a function of arterial oxygen levels and cardiac output.
This implies that both ventilation and cardiac function are crucial for patient survival.
Positive end-expiratory pressure (PEEP) mechanical ventilation is essential to ensure adequate arterial oxygenation in patients with ARDS.
Positive pressure ventilation, however, can, in conjunction with improved oxygenation, reduce cardiac output (see below). The improvement in arterial oxygenation is of little or no use if the simultaneous increase in intrathoracic pressure induces a corresponding reduction in cardiac output.
Consequently, the maximum level of PEEP tolerated by the patient is generally dependent on cardiac function.
Severe ARDS can result in death due to tissue hypoxia when maximal fluid therapy and vasopressor agents do not adequately improve cardiac output for the given level of PEEP necessary to ensure efficient pulmonary gas exchange.
In the most severe patients, and particularly those undergoing mechanical ventilation, a state of malnutrition often results.
The effects of malnutrition on the lungs include: immunosuppression (reduced macrophage and T-lymphocyte activity), attenuated respiratory stimulation by hypoxia and hypercapnia, impaired surfactant function, reduced intercostal and diaphragm muscle mass, decreased respiratory muscle contraction force, in relation to the body’s catabolic activity, thus malnutrition can influence many critical factors, not only for the effectiveness of maintenance and supportive therapy, but also for weaning from mechanical ventilator.
If practicable, enteral feeding (administration of food via a nasogastric tube) is preferable; but if intestinal function is compromised, parenteral (intravenous) feeding becomes necessary to infuse the patient with sufficient protein, fat, carbohydrates, vitamins and minerals.
Mechanical ventilation in ARDS
Mechanical ventilation and PEEP do not directly prevent or treat ARDS but, rather, keep the patient alive until the underlying pathology is resolved and adequate lung function is restored.
The mainstay of continuous mechanical ventilation (CMV) during ARDS consists of conventional ‘volume-dependent’ ventilation using tidal volumes of 10-15 ml/kg.
In the acute phases of the disease, full respiratory assistance is used (usually by means of ‘assist-control’ ventilation or intermittent forced ventilation [IMV]).
Partial respiratory assistance is usually given during recovery or weaning from the ventilator.
PEEP can lead to the resumption of ventilation in atelectasis zones, transforming previously shunted lung areas into functional respiratory units, resulting in improved arterial oxygenation at a lower fraction of inspired oxygen (FiO2).
Ventilation of already atelectatic alveoli also increases functional residual capacity (FRC) and lung compliance.
Generally, the goal of CMV with PEEP is to achieve a PaO2 greater than 60 mmHg at a FiO2 of less than 0.60.
Although PEEP is important for maintaining adequate pulmonary gas exchange in patients with ARDS, side effects are possible.
Reduced lung compliance due to alveolar overdistension, reduced venous return and cardiac output, increased PVR, increased right ventricular afterload, or barotrauma may occur.
For these reasons, ‘optimal’ PEEP levels are suggested.
The optimal PEEP level is generally defined as the value at which the best O2del is obtained at a FiO2 below 0.60.
PEEP values that improve oxygenation but significantly reduce cardiac output are not optimal, because in this case O2del is also reduced.
The partial pressure of oxygen in mixed venous blood (PvO2) provides information on tissue oxygenation.
A PvO2 below 35 mmHg is indicative of suboptimal tissue oxygenation.
A reduction in cardiac output (which can occur during PEEP) results in low PvO2.
For this reason, PvO2 can also be used for the determination of optimal PEEP.
Failure of PEEP with conventional CMV is the most frequent reason for switching to ventilation with an inverse or high inspiratory/expiratory (I:E) ratio.
Reverse I:E ratio ventilation is currently practised more often than high-frequency ventilation.
It provides better results with the patient paralysed and the ventilator timed so that each new respiratory act starts as soon as the previous exhalation has reached the optimal PEEP level.
The respiratory rate can be reduced by prolonging inspiratory apnoea.
This often leads to a reduction in mean intrathoracic pressure, despite the increase in PEEP, and thus induces an improvement in O2del mediated by an increase in cardiac output.
High-frequency positive pressure ventilation (HFPPV), high-frequency oscillation (HFO), and high-frequency ‘jet’ ventilation (HFJV) are methods that are sometimes able to improve ventilation and oxygenation without resorting to high lung volumes or pressures.
Only HFJV has been widely applied in the treatment of ARDS, without significant advantages over conventional CMV with PEEP being conclusively demonstrated.
Membrane extracorporeal oxygenation (ECMO) was studied in the 1970s as a method that could guarantee adequate oxygenation without resorting to any form of mechanical ventilation, leaving the lung free to heal from the lesions responsible for ARDS without subjecting it to the stress represented by positive pressure ventilation.
Unfortunately, patients so severe that they did not respond adequately to conventional ventilation and were therefore eligible for ECMO, had such severe lung lesions that they still underwent pulmonary fibrosis and never recovered normal lung function.
Weaning off mechanical ventilation in ARDS
Before taking the patient off the ventilator, it is necessary to ascertain his or her chances of survival without respiratory assistance.
Mechanical indices such as maximum inspiratory pressure (MIP), vital capacity (VC), and spontaneous tidal volume (VT) assess the patient’s ability to transport air in and out of the chest.
None of these measures, however, provide information on the resistance of the respiratory muscles to work.
Some physiological indices, such as pH, dead space to tidal volume ratio, P(A-a)O2, nutritional status, cardiovascular stability, and acid-base metabolic balance reflect the patient’s general condition and his ability to tolerate the stress of weaning from the ventilator.
Weaning from mechanical ventilation occurs progressively, to ensure that the patient’s condition is sufficient to ensure spontaneous breathing, before removing the endotracheal cannula.
This phase usually begins when the patient is medically stable, with a FiO2 of less than 0.40, a PEEP of 5 cm H2O or less and the respiratory parameters, referred to earlier, indicate a reasonable chance of resumption of spontaneous ventilation.
IMV is a popular method for weaning patients with ARDS, because it allows the use of a modest PEEP until extubation, allowing the patient to gradually cope with the effort required for spontaneous breathing.
During this weaning phase, careful monitoring is important to ensure success.
Changes in blood pressure, increased heart or respiratory rate, reduced arterial oxygen saturation as measured by pulse oximetry, and worsening mental functions all indicate failure of the procedure.
A gradual slowing down of weaning may help prevent a failure related to muscle exhaustion, which may occur during the resumption of autonomous breathing.
Monitoring during ARDS
Pulmonary arterial monitoring allows cardiac output to be measured and O2del and PvO2 to be calculated.
These parameters are essential for the treatment of possible haemodynamic complications.
Pulmonary arterial monitoring also allows measurement of right ventricular filling pressures (CVP) and left ventricular filling pressures (PCWP), which are useful parameters for determining optimal cardiac output.
Pulmonary arterial catheterisation for haemodynamic monitoring becomes important in the event that blood pressure falls so low as to require treatment with vasoactive drugs (e.g. dopamine, norepinephrine) or if pulmonary function deteriorates to the point where PEEP of more than 10 cm H2O is required.
Even the detection of a pressor instability, such as to require large fluid infusions, in a patient who is already in a precarious cardiac or respiratory condition, may require the placement of a pulmonary artery catheter and haemodynamic monitoring, even before vasoactive drugs need to be administered.
Positive pressure ventilation may alter haemodynamic monitoring data, leading to a fictitious increase in PEEP values.
High PEEP values can be transmitted to the monitoring catheter and be responsible for an increase in the calculated CVP and PCWP values that does not correspond to reality (43).
This is more likely if the catheter tip is located near the anterior chest wall (zone I), with the patient supine.
Zone I is the non-declivity lung area, where the blood vessels are minimally distended.
If the end of the catheter is located at the level of one of them, the PCWP values will be greatly influenced by the alveolar pressures, and will therefore be inaccurate.
Zone III corresponds to the most declivous lung area, where the blood vessels are almost always distended.
If the end of the catheter is located in this area, the measurements taken will be only very marginally affected by ventilation pressures.
Placement of the catheter at the level of zone III can be verified by taking a lateral projection chest X-ray, which will show the catheter tip below the left atrium.
Static compliance (Cst) provides useful information on lung and chest wall stiffness, while dynamic compliance (Cdyn) assesses airway resistance.
Cst is calculated by dividing tidal volume (VT) by static (plateau) pressure (Pstat) minus PEEP (Cst = VT/Pstat – PEEP).
Pstat is calculated during a short inspiratory apnoea after a maximal breath.
In practice, this can be achieved by using the pause command of the mechanical ventilator or by manual occlusion of the expiratory line of the circuit.
Pressure is checked on the ventilator manometer during apnoea and must be below the maximum airway pressure (Ppk).
Dynamic compliance is calculated in a similar way, although in this case Ppk is used instead of static pressure (Cdyn = VT/Ppk – PEEP).
Normal Cst is between 60 and 100 ml/cm H2O and can be reduced to around 15 or 20 ml/cm H20 in severe cases of pneumonia, pulmonary oedema, atelectasis, fibrosis and ARDS
Since a certain pressure is required to overcome airway resistance during ventilation, part of the maximum pressure developed during mechanical respiration represents the flow resistance encountered in the airways and ventilator circuits.
Thus, Cdyn measures the overall impairment of airway flow due to changes in both compliance and resistance.
Normal Cdyn is between 35 and 55 ml/cm H2O, but can be adversely affected by the same diseases that reduce Cstat, and also by factors that can change resistance (bronchoconstriction, airway oedema, retention of secretions, airway compression by a neoplasm).
Read Also:
Emergency Live Even More…Live: Download The New Free App Of Your Newspaper For IOS And Android
Obstructive Sleep Apnoea: What It Is And How To Treat It
Obstructive Sleep Apnoea: Symptoms And Treatment For Obstructive Sleep Apnoea
Our respiratory system: a virtual tour inside our body
Tracheostomy during intubation in COVID-19 patients: a survey on current clinical practice
FDA approves Recarbio to treat hospital-acquired and ventilator-associated bacterial pneumonia
Clinical Review: Acute Respiratory Distress Syndrome
Stress And Distress During Pregnancy: How To Protect Both Mother And Child
Respiratory Distress: What Are The Signs Of Respiratory Distress In Newborns?
Sepsis: Survey Reveals The Common Killer Most Australians Have Never Heard Of
Sepsis, Why An Infection Is A Danger And A Threat To The Heart